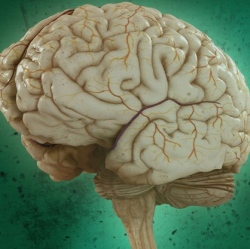
A technique that switches brain circuits on and off has taken neuroscience by storm and is now undergoing a new round of innovation. Optogenetics refers to techniques that use genetic modification of cells so they can be manipulated with light. The net result is a switch that can turn brain cells off and on like a bedside lamp.
The technique has enabled neuroscientists to achieve previously unimagined feats and two of its inventors, Karl Deisseroth of Stanford University and the Howard Hughes Medical Institute and Ed Boyden of Massachusetts Institute of Technology, received a Breakthrough Prize in the life sciences on November 8 in recognition of their efforts. The technology is able to remotely control motor circuits, one example is having an animal run in circles at the flick of a switch. It can even label and alter memories that form as a mouse explores different environments. These types of studies allow researchers to firmly establish a cause-and-effect relationship between electrical activity in specific neural circuits and various aspects of behavior and cognition, making optogenetics one of the most widely used methods in neuroscience today.
Generally, optogenetics refers to any method for communicating with cells using genetics plus optics, and that can mean observing cell activity, not just turning a neuron on or off. Nongenetic approaches, such as fluorescent dyes that increase cell illumination in response to activity, have been used previously, but lack the precision of targeting a particular type of cell.
A new way to watch what’s happening with cells utilizes the same genetic targeting methods used to switch circuits off and on, Except now indicator proteins are integrated into selected cells via genetic tweaks. The indicators generally consist of a protein sensitive to cell activity, linked to a fluorescent protein, so they light up in response to a cell’s firing. Combining these genetically targeted optical readouts with the standard armamentarium of tools for controlling cell activity unlocks the full potential of optogenetics. The combined technique allows researchers to hold two-way conversations with populations of selected neurons, using only pulses of light. Once various technical difficulties are overcome, researchers will be able to carry out this dialogue with single neurons, in real-time, allowing a level of interaction with awake, functioning brains, that has not been possible before now.
At the recent Society for Neuroscience meeting in Chicago, several leading researchers spoke about “all-optical interrogation of neural circuits” and co-wrote an accompanying review in The Journal of Neuroscience. They outlined the challenges involved and described pioneering work to overcome these obstacles. The approach has the potential to shed light, in literal terms, on the relationship between brain activity, on one hand, and cognition, behavior and emotion, on the other. This goal fits well with the U.S. BRAIN initiative, which aims to encourage the development of new tools for exploring the link between neural signals and cognition.
All-optical techniques could revolutionize neuroscience by allowing researchers to simultaneously control and monitor precisely even single spikes from either single neurons or large ensembles of neurons as experimental animals move freely about. "This approach will open a whole new range of experiments," says Michael Hausser, a neuroscientist at University College London, who co-chaired the conference session alongside Emiliani. “Unlocking the full potential of optogenetics requires going beyond targeting genetically-defined cell-types, to targeting cells according to functional properties, rather than just genetic identity.”
In other words, rather than just passively monitoring, or observing the results of an experiment in which the level of stimulation of neurons must be carefully planned beforehand, these innovations will enable researchers to adapt how they stimulate cells depending on how cells are behaving. “If you can tailor stimulation to activity patterns, you can do the manipulation on the fly,” Hausser explains. “For instance, during a decision-making experiment, if you can track the activity of ensembles of neurons in real time, you can influence behavior more effectively by manipulating ensembles as they form.”
“These are two game-changing advances, compared to what we could do before: targeting functional ensembles and manipulating activity in real time,” Hausser says. “Ultimately these approaches could help define the neural codes used in the brain to drive behavior.”