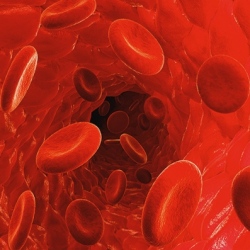
The ability to transform one cell type into another has been demonstrated for many combinations of types in the laboratory, if not with the reliability needed to move on to clinical therapies. The types of interest here are the fibroblasts that form scar tissue and the cardiomyocytes of heart muscle.
Heart tissue is not very regenerative, and scarring and reduced function follows injury of any sort, especially those arising from the structural failures of age: heart attacks and other forms of cardiovascular disease that can deprive the heart of oxygen and nutrients. With the growing damage of aging it is also the case that regeneration runs awry.
The presence of senescent cells and chronic inflammation results in fibrosis, the generation of scar-like collagen structures and raised numbers of fibroblasts rather than the correct maintenance of healthy muscle tissue. What if those fibroblasts could be converted in situ into cardiomyocytes, however?
Quote:
Reversing scar tissue after a heart attack to create healthy heart muscle: this would be a game-changer in the field of cardiology and regenerative medicine. In the lab, scientists have shown it’s possible to change fibroblasts (scar tissue cells) into cardiomyocytes (heart muscle cells), but sorting out the details of how this happens hasn’t been easy, and using this kind of approach in clinics or even other basic research projects has proven elusive.
Now, researchers have used single cell RNA sequencing technology in combination with mathematical modeling and genetic and chemical approaches to delineate the step-by-step molecular changes that occur during cell fate conversion from fibroblast to cardiomyocyte. The scientists not only successfully reconstructed the routes a single cell could take in this process but also identified underlying molecular pathways and key regulators important for the transformation from one cell type to another.
"We used direct cardiac reprogramming as an example in this study. But the pipelines and methods we’ve established here can be used in any other reprogramming process, and potentially other unsynchronized and heterogeneous biological processes." When we are babies, embryonic stem cells throughout our bodies gradually change into a variety of highly specialized cell types, such as neurons, blood cells, and heart muscle cells.
For a long time, scientists thought these specific cell types were terminal; they could not change again or be reverted back to a state between embryonic and their final differentiated stage. Recent discoveries, though, show it’s possible to revert terminally differentiated somatic cells to a pluripotent state – a kind of "master" cell that can self-produce and potentially turn into any kind of cell in the body. Scientists have also figured out how to convert one kind of differentiated somatic cell type into another without detouring through the pluripotent stage or the original progenitor stage.
Direct cardiac reprogramming, a promising approach for cardiac regeneration and disease modeling, involves direct conversion of cardiac non-myocytes into induced cardiomyocytes (iCMs) that closely resemble endogenous CMs. Like any reprogramming process, the many cells that are being reprogrammed don’t do so at the same time. "So, at any stage, the cell population always contains unconverted, partially reprogrammed, and fully reprogrammed cells, which makes it difficult to study using traditional approaches.
Some of what we found is clinically important. For example, we know that after a heart attack, cardiac fibroblasts around the injured area are immediately activated and become highly proliferative but this proliferative capacity decreases over time. A way to take advantage of the varied cell cycle status of fibroblasts over the progression of a heart attack and its aftermath would certainly broaden the application of cellular reprogramming for patients and optimize outcomes."
The team continued with detailed functional analysis of the top candidate – the splicing factor called Ptbp1. Evidence suggests it as a critical barrier to the acquisition of cardiomyocyte-specific splicing patterns in fibroblasts. The study showed that Ptbp1 depletion promoted the formation of more iCMs. "The new knowledge learned from our mechanistic studies of how a single splicing factor regulates the fate conversion from fibroblast to cardiomyocyte is really a bonus to us. Without the unbiased nature of this approach, we would not gain such fresh, valuable information about the reprogramming process. And that’s the beauty of our platform."