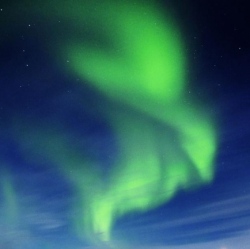
Daylight savings notwithstanding, time always ticks forward. But why not backward? Why do we remember the past and not the future? For a group of physicists, the answers to these deep and complex questions may arise from a familiar source: gravity.
Even though time is such a fundamental part of our experience, the basic laws of physics don’t seem to care in which direction it goes. For example, the rules that govern the orbits of planets work the same whether you go forward or backward in time. You can play the motions of the solar system in reverse and they look completely normal; they don’t violate any laws of physics. So what distinguishes the future from the past?
“The problem of the arrow of time has been boggling minds forever,” said Flavio Mercati of the Perimeter Institute for Theoretical Physics in Waterloo, Canada. Most people who’ve thought about this arrow of time say it’s determined by entropy, the amount of disorder in a system (like, say, a bowl of cereal, or the universe). According to the second law of thermodynamics, the overall entropy of a closed system must always increase. And time appears to travel in the same direction as rising entropy.
When an ice cube in your glass melts and dilutes your lemonade, for instance, entropy increases. When you scramble an egg, entropy increases. Both of these examples are irreversible: you can’t freeze a water ice cube out from your lemonade or unscramble an egg. The sequence of events, and thus time, goes in only one direction. If time’s arrow follows the increase of entropy, and if the entropy in the universe is always increasing, then it means that at some point in the past, entropy must have been low. Therein lies the puzzle: why was the universe in such a low entropy state in the first place?
According to Mercati and his colleagues, there was no special, initial state at all. Instead, a state that gets time pointing forward arises naturally from a universe dictated by gravity. The researchers make this argument in a paper recently published in the journal Physical Review Letters. To test their idea, they simulated the universe as a collection of 1,000 particles that interact with one another only by gravity, representing the galaxies and stars that float around the cosmos.
The researchers found that regardless of starting positions and velocities, at some point the particles inevitably find themselves clustered together in a ball before dispersing again. This moment of clumping is equivalent to the Big Bang, when the whole universe was squeezed into an infinitesimally small point. Instead of using entropy, the researchers describe their system with a quantity they call complexity, which they define as roughly the ratio of the distance between the two particles farthest from each other to the distance between the two particles closest to each other. When the particles are clumped together, complexity is at its lowest.
The key idea, Mercati explains, is that this moment of lowest complexity arises naturally from the group of gravitationally interacting particles, no special initial conditions are needed. Complexity then increases as the particles disperse, representing the expansion of the universe and the forward progress of time.