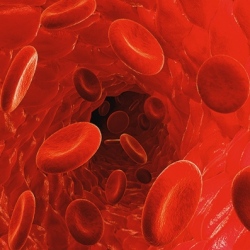
Advances in gene editing technologies are enabling researchers to understand far more about the contribution of genetics to disease. The technique involves the insertion, deletion or replacement of DNA in the genome of an organism using engineered nucleases known as molecular scissors.
Building on the success of past studies and programmes such as the Human Genome Project, scientists are continuing to use DNA sequencing to search for mutations or genetic variations associated with the development or progression of a disease.
The inability of existing therapeutics to treat diseases such as cancers in certain people has led to experimental gene therapies on humans. For instance, although the standard treatment of chemotherapy followed by bone marrow transplantation is commonly used in children with leukaemia, success rates for infants are only 25 per cent.
Low success rates like this one prompt scientists to find new ways of creating personalised treatments for patients who may otherwise be faced with no viable treatment options.
In 2015, the unique case of one-year-old acute lymphoblastic leukaemia patient Layla, who was saved by pioneering gene editing research, broke new ground. Professor of Cell and Gene Therapy at UCL, Waseem Qasim, worked with his team to provide a personalised treatment option for Layla using a TALEN-based approach. They introduced T cells from a healthy donor and disabled genes that would attack them in her body with molecular scissors.
“We’ve known for some time that some types of leukaemia can be eliminated by powerful immune effects mediated by donor T cells after a bone marrow transplant,” elaborates Qasim. “More recently, it has become possible to add anti-leukemic chimeric antigen receptors (CARs) to a patient’s own T cells, which are then re-infused and can clear leukaemia.”
The challenge of administering these therapies to small infants arises because of the difficulty involved in collecting enough healthy T cells to use, which is why the team at UCL decided to explore the use of donor cells. “As well as adding a CAR, we made additional edits to two important genes that would allow the cells to be given to any recipient,” Qasim explains.
“To do this, we disabled the T cell’s own receptor so that they don’t recognise the recipient as foreign.” The researchers also removed a molecule called CD52 to make the cells ‘invisible’ to a powerful antibody drug to prevent rejection of the cells by the patient.
A month after Layla received the experimental treatment, the T cells had destroyed all of the cancerous cells in her bone marrow. Although she can only be given the all clear in a year or two, the signs are promising.
Further T cell research is being carried out across UCL, including work by Dr Martin Pule on the development of new CARs for different kinds of cancer. Many of these could be developed further using editing approaches to create a ready-made bank of cells available to patients as soon as they are required.
The early success of gene editing treatments could lead to them becoming mainstream options in the clinic in the not-to-distant future. “In the near term, I would expect more applications using T cells, including formal trials of universal CAR T cells for leukaemia,” says Qasim.
“Beyond that, we should see attempts to correct blood stem cells in inherited immune diseases, and perhaps other blood conditions. Correction of cells directly in patients is still some years away, but it’s a fast moving area and the reagents are evolving rapidly.”